SVTg — The Key to Unlocking a Joyful Journey
According to literature, inhibiting the LHb can induce a reward experience. The authors tested the effect of optogenetic stimulation of SVTg in behavioral experiments. In vGAT-Cre mice, AAV expressing ChR2 was injected into the LHb, targeting SVTg → LHb neurons, and optical fibers were implanted above the SVTg (Figure 3A). Real-time place preference (RTPP) and conditioned place preference (CPP) tests (Figure 3B) showed that, unlike CTRL mice, ChR2 mice demonstrated a strong immediate preference for the box that received stimulation during the RTPP test under both 4Hz and 20Hz light stimulation. In the CPP test, ChR2 mice previously exposed to 20Hz stimulation showed a preference for the box where the stimulation had occurred (Figure 3, C and D); during the adaptation period, the mice showed no preference for either chamber (Figure 3C). To confirm that the SVTg fibers targeting the LHb were directly responsible for inducing place preference behavior, the authors conducted a similar experiment but only stimulated the SVTg fibers in the LHb (Figure 3E) and performed the same RTPP and CPP tests as above. Similarly, the experiment found that, compared to CTRL mice, ChR2 mice showed strong RTPP and CPP for the box that had received stimulation (Figure 3F). This suggests that activation of SVTg can trigger positive reward experiences in mice, playing a role in transmitting and converting reward signals into behavioral preferences.
Figure 3: SVTg neurons induce rewarding experience in mice
To test whether mice would actively activate SVTg neurons, the authors conducted an optogenetic self-stimulation experiment. AAV expressing ChR2 was injected into the LHb of vGAT-Cre mice for retrograde tracing, infecting SVTg→LHb neurons, and an optical fiber was implanted above the SVTg (Figure 4G). Mice on restricted diets underwent 10 days of training in an operant conditioning task, where they would receive light stimulation only when they poked their nose into a predefined (ILLUM) hole (Figure 4H). The results showed that, compared to CTRL mice, ChR2 mice more frequently poked their noses into the ILLUM hole (Figure 4I). When tested for memory of preference for the ILLUM hole without light stimulation, ChR2 mice still showed a stronger preference for the ILLUM hole than CTRL mice (Figure 4, I and J). These results suggest that activation of SVTg has a rewarding effect, and that mice will actively stimulate their own SVTg, indicating that SVTg may play a role in driving reward-seeking behavior.
To investigate whether optogenetic activation of SVTg could reduce anxiety in mice, ChR2 and CTRL mice were placed in an elevated plus maze (EPM), where they received continuous 4 Hz light stimulation for 10 minutes throughout the experiment (Figure 4, K and L). Compared to CTRL mice, ChR2 mice spent more time on the open arms and entered the open arms more frequently (Figure 4M), suggesting that activation of SVTg may have anxiolytic effects.
Figure 4: Mice can self-stimulate SVTg and exhibit anxiolytic effects.
Dopamine release in the nucleus accumbens (NAc) can transmit signals of reward experience. Although SVTg does not directly project to the NAcc (Figure 2Q), the authors investigated whether SVTg can modulate reward behavior via the known LHb→VTA→NAcc pathway. AAV expressing ChR2 was injected into vGAT-Cre mice to infect SVTg→LHb neurons, while another AAV expressing a dopamine indicator (DA4.4-GRABDA) was used to target the NAcc, with optical fibers implanted in the SVTg for optogenetic stimulation and dopamine release recording. The experiment found that activation of SVTg can also trigger dopamine release in the NAcc (Figure 5A-5C), with release levels similar to those under water reward stimulation (Figure 5C). These results further confirm that SVTg can mediate reward responses by modulating dopamine.
To test whether natural rewards trigger immediate early gene expression in SVTg neurons, the authors examined c-Fos expression in SVTg neurons following food reward. First, vGAT-Cre mice were infected with an AAV expressing GFP in the SVTg→LHb neurons. Mice on a restricted diet were divided into two groups: one group received sucrose reward pellets in a novel environment (REW mice), while the other group was placed in the same environment without reward pellets (CTRL mice) (Figure 5D). The results showed that, compared to CTRL mice, reward-induced REW mice exhibited increased c-Fos activity in the SVTg (Figure 5, E and F). Calcium imaging experiments revealed that water rewards in trained, head-fixed, and water-restricted mice also induced increased activity in SVTg neurons (Figure 5G-5J).
To investigate whether expectation of reward activates SVTg neurons, the same mouse groups were used, where SVTg→LHb neurons expressed GCaMP8m. It was found that neutral stimuli (such as pure tones or short/long environmental light) did not activate the SVTg neurons in head-fixed mice (Figure 5K-5M). On the first day of subsequent experiments, water-restricted mice were trained to receive a drop of water at the end of a 2-second tone (Figure 5K). On the second day, it was found that the tone predicting the reward increased activity in SVTg neurons, without affecting the control signal (Figure 5, L and M). These results further suggest that SVTg activity is related to reward prediction.
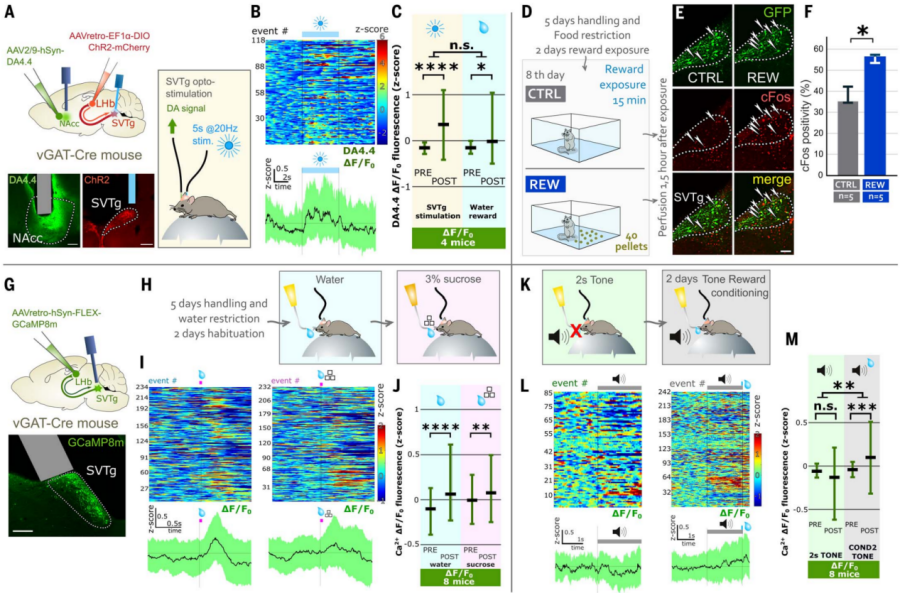
Figure 5: SVTg induces dopamine release in the NAc and is activated by reward experience.
SVTg—The "Loyal Guardian" of Memory
To remember where food was found previously, the "reward" feeling must be linked to the location of the food. To test the role of SVTg in these processes, the authors used a food-induced location preference paradigm. AAV expressing the inhibitory optogenetic protein stGtACR2 was used to retrogradely infect SVTg→LHb neurons, with an optical fiber implanted above the SVTg (Figure 6A). After habituation and food restriction, mice were placed in a two-chamber box for 6 days, where neither CTRL nor stGtACR2 mice showed location preference during the habituation period (Figure 6, B and C). On days 7 and 8, mice were given 40 sucrose pellets in one of the boxes, with light stimulation (Figure 6B). The results showed that, compared to CTRL mice, stGtACR2 mice had a lower preference for the location with the pellets. On day 9, neither the pellets nor light stimulation were given, and the results showed that CTRL mice preferred the location where food had been, while stGtACR2 mice did not (Figure 6, C and D). This suggests that normal SVTg activity is necessary for reward location memory.
The authors further investigated whether SVTg activity is essential for maintaining the balanced value circuit through optogenetic inhibition. The results showed that after light stimulation of SVTg, stGtACR2 mice immediately exhibited real-time place aversion (RTPA) and conditioned place aversion (CPA) (Figure 6F-6H). These results suggest that SVTg may have baseline neuronal activity that maintains healthy value detection and motivational states during spatial exploration.
Figure 6: Inhibition of SVTg disrupts food-induced preference learning and induces aversion.
SVTg—The "Master of Emotional Balance"
In addition, the authors also investigated the role of SVTg in balancing fear responses. Using calcium imaging technology, they recorded SVTg neuronal activity during aversive events (Figure 7, A and B). The results showed that aversive air puffs rapidly activated SVTg neurons (Figure 7C-7E). Further, after 10 random air puff stimuli, it was found that the initial five air puffs triggered stronger SVTg activation compared to the last five (Figure 7F-7H). Additionally, the first three sets of air puffs induced stronger SVTg activation than the last three (Figure 7I). These results suggest that rapid adaptive changes occurred in the SVTg-related circuits or within the SVTg neurons themselves, with a stronger response to early stimuli that gradually weakened over time.
To investigate whether this rapid natural activation of SVTg neurons is necessary to prevent excessive fear responses, the authors tested how mice responded to fear when SVTg neuronal activity was inhibited. AAV expressing stGtACR2 was used to infect SVTg→LHb neurons in vGAT-Cre mice, with optical fibers implanted above the SVTg (Figure 5J). The results showed that inhibiting SVTg during a footshock test significantly increased fear responses (freezing time) in both the original context (CTX-A) and a novel context (CTX-B). However, activating SVTg inhibited fear responses in the original context (CTX-A) (Figure 7, J and K). These data suggest that SVTg balances fear responses, controls the strength of fear memory formation, and prevents abnormal excessive generalization.
Figure 7: SVTg neurons are activated by aversive events to control the formation of fear memories.
SVTg's "Higher Commanders"
To determine which brain regions target SVTg neurons through direct synaptic connections, the authors used monosynaptic rabies virus (RV) tracing technology. In vGAT-Cre mice, Cre-dependent helper viruses were used to infect SVTg neurons, and retrograde RV was injected into the LHb of the same mouse (Figure 8, A and B). The results showed that several key brain regions encoding value, motivation, and aversive emotions targeted SVTg GABAergic neurons through monosynaptic connections, including the median raphe nucleus (MRR), which plays a foundational role in aversive behavior. Additionally, several higher-order associative cortical regions, such as the orbitofrontal cortex (OFC) and anterior cingulate cortex (ACC), were also found to target SVTg neurons (Figure 8, C and D).
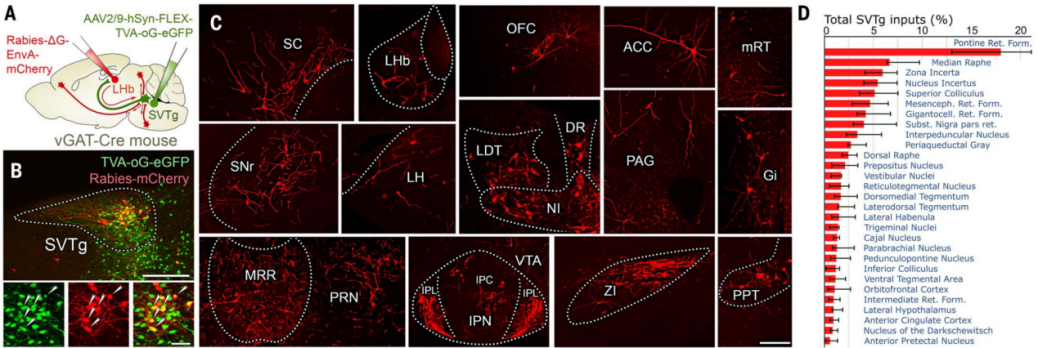
Figure 8: Monosynaptic tracing of upstream brain regions targeting SVTg neurons.
The previous behavioral and tracing experiment results suggest that reward prediction activation of SVTg may be regulated by higher-order associative cortices. To further confirm this, the authors retrogradely infected cortical neurons on the SVTg side of vGluT1-Cre mice (Figure 9, E and F). The results showed that the largest number of pyramidal cells was retrogradely labeled in the orbitofrontal cortex (OFC), while other higher-order associative cortices, including the ACC and other prefrontal cortex regions, also targeted SVTg (Figure 9G). Immunohistochemical optical and electron microscopy imaging confirmed that the OFC targets the dendrites and cell bodies of SVTg neurons via excitatory axon terminals, suggesting that SVTg may play a direct role in the reward-related functions executed by the higher-order OFC.
AAV expressing ChR2 was injected into the OFC of vGluT1-Cre mice, and optical fibers were implanted above the SVTg for location preference and fear conditioning experiments (Figure 9H). The results showed that stimulating the OFC at the SVTg axon terminals, ChR2 mice displayed a preference for the stimulated side in the RTPP and CPP tests (Figure 9I). Additionally, in the fear conditioning experiment, activation of OFC fibers reduced the fear response in ChR2 mice in the CTX-A context (Figure 9J-9K). These experiments suggest that the OFC may play a role in value processing through SVTg.
Figure 9: SVTg mediates reward processing induced by the OFC
The "genetic code" and unique "personality" of SVTg
By analyzing the input neurotransmitter systems of SVTg neurons, a better understanding of the role of SVTg has been achieved. Using single-cell mRNA sequencing, the authors characterized the entire mRNA library of SVTg GABAergic neurons (Figure 10A). The results showed that SVTg neurons expressed GABAergic neuron markers (GAD65, GAD67, GAT1, and vGAT) as well as various reward-related receptor genes. They also highly expressed reelin (Reln), calcium channel 1G (Cacna1g), and specific transcription factor genes (Satb1, Pax6) (Figures 10B-10D). Further immunostaining revealed that almost all SVTg neurons throughout the entire head-to-tail range of this nucleus in the midbrain expressed Satb1, Pax6, and Reln, with Satb1 being the best candidate marker to identify SVTg in the pons (Figure 10D). In vitro electrophysiological recordings showed that all SVTg neurons could produce burst firing, and their activity was controlled by cortical and subcortical glutamatergic input (Figures 10E-10G). Serotonin exerted a dual excitatory effect on SVTg neurons, mediated by at least two independent pathways. Additionally, SVTg neurons received innervation from serotonergic axon fibers, some of which formed classic synapses (Figure 10F).
Figure 10 Gene expression profile and in vitro electrophysiological properties of SVTg neurons.
The evolutionary "footprint" of SVTg
The pons, a part of the brainstem, is considered to be a very ancient and highly conserved region in evolutionary terms, and therefore its neuronal populations are likely present in the brains of most mammals, possibly even more evolved. Given that Satb1 exhibits high specificity in the SVTg neurons of the mouse brainstem (Figure 10D), the authors used it to locate SVTg in the pons of rats, rhesus macaques (Macaca mulatta), and humans. The results showed that Satb1-positive SVTg neurons were found in the brainstem of rats, rhesus macaques, and humans, located below the fourth ventricle, between LDTg, LC, and DR neurons, indicating that SVTg is highly conserved in evolution (Figure 11).
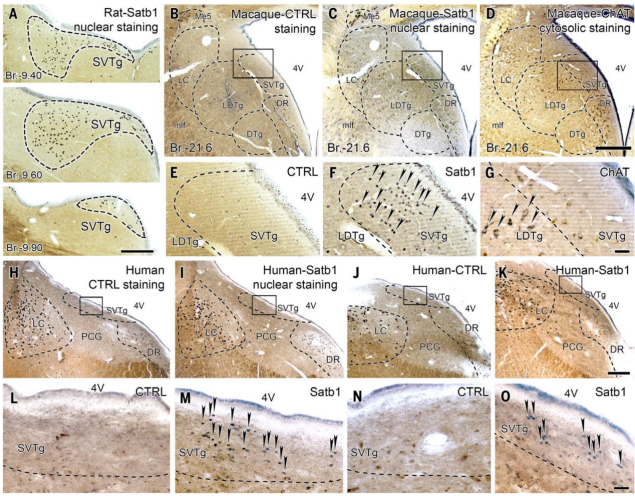
Figure 11 Satb1-positive SVTg neurons were found in the dorsal pons of rats, monkeys, and humans.
Significance of this study
- Discovery of a new brainstem reward center: This study is the first to identify and name the brainstem nucleus located below the fourth ventricle, known as SVTg, and confirm its role as a GABAergic inhibitory nucleus that plays a key role in regulating reward processing and emotional value assessment.
- Revealing the functional role of SVTg: Through various experimental methods, the study confirmed that SVTg, under the activation of reward and reward-predicting cues, promotes positive emotional states, reduces anxiety, and regulates reward-related dopamine release by inhibiting the LHb.
- Elucidating the neural circuitry of SVTg: The study provides a detailed description of the connections of SVTg with multiple brain regions, including its direct inhibitory effect on the LHb and interactions with areas such as the prefrontal cortex and nucleus accumbens, revealing its complex neural network in emotional and motivational regulation.
- Potential clinical applications: The findings suggest that SVTg may play an important role in emotional disorders such as anxiety and depression, as well as in addictive behaviors, providing new targets for future therapeutic approaches, such as alleviating related symptoms by modulating SVTg activity.
At the end, include a small anecdote
In the field of neuroscience research, commonly used AAV serotypes such as 1, 2, 5, 6, 8, and 9 have been widely utilized. However, their inefficient retrograde transduction capabilities have limited their application in studying projection networks or treating diseases. Particularly when investigating specific neuronal inputs to a nucleus, there is a need for low-toxicity, easily producible retrograde-transducing viruses. Scientists have developed a mutant of AAV2, known as AAV2-retro, through directed evolution. This serotype enables efficient retrograde labeling of neural circuits in the mouse brain, with retrograde tracing efficiency comparable to traditional tracers.
However, Professor Xu Fuqiang's team discovered that AAV2-retro has low infection efficiency in certain nuclei, such as the caudate putamen (CP), nucleus accumbens (ACB), substantia innominata, bed nucleus of the stria terminalis, lateral hypothalamic area (LHA), lateral preoptic area, substantia nigra pars reticulata, superior colliculus (related to motor function), and raphe nuclei. For example, in CP and ACB, which are rich in GABAergic neurons and directly connected to the ventral tegmental area (VTA), almost no cell bodies were labeled by AAV2-retro (Fig12).
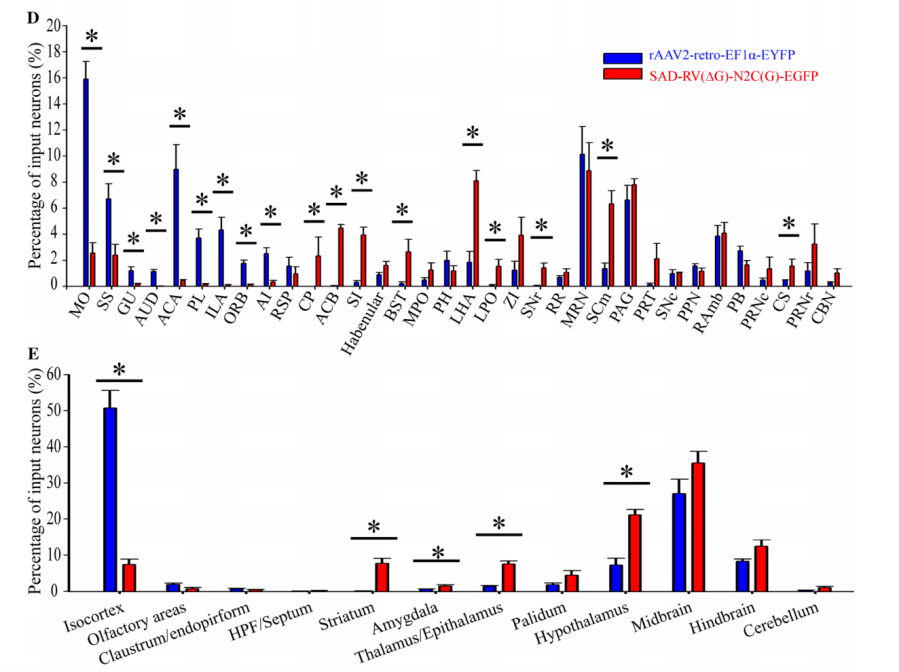
Figure 12 Retrograde infection tropism biases of SAD-RV(DG)-N2C(G) and rAAV2-retro injected into the VTA. Zhu X, Lin K, Liu Q, et al, [J]. Neuroscience bulletin Ref 1
Gabor Nyiri and Szabolcs Káli's team found that using AAV2-retro made it difficult to verify whether SVTg→LHb neurons could directly inhibit neurons projecting to the VTA. As mentioned earlier, AAV2-retro has low efficiency in infecting specific types of neuronal terminals in certain nuclei. Gabor’s team tried a novel retrograde-transducing serotype, AAV11.. Ultimately, they confirmed that GABAergic neurons in the SVTg→LHb pathway transmit negative valence signals to the VTA, uncovering a new neural circuit.
The application of AAV11 for retrograde tracing is the result of extensive screening by Professor Xu Fuqiang's team. It serves as a powerful complement to AAV2-retro, offering a robust tool for retrograde viral tracing. When combined with fiber photometry, AAV11 can deliver calcium-sensitive indicators retrogradely, enabling the monitoring of neuronal activity in functional networks under the control of neuron-specific promoters or the Cre-lox system. Additionally, AAV11 equipped with the GfaABC1D promoter exhibits superior astrocyte tropism in vivo compared to AAV8 and AAV5. When combined with bidirectional multi-vector axon-astrocyte labeling, AAV11 can be used to study neuron-astrocyte interactions (Reference 2, linked to the tweet on AAV11 astrocyte infection). Moreover, the team demonstrated high transduction specificity of AAV11 for microglia in various brain regions and the spinal cord using the IBA1 promoter and miRNA-9 targeting sequences (Reference 3, linked to the original tweet on AAV11 microglia targeting). These properties make AAV11 a promising tool for mapping and manipulating neural circuits and for gene therapy in neurological and neurodegenerative disorders.
References:
1. Zhu X, Lin K, Liu Q, et al. Rabies virus pseudotyped with CVS-N2C glycoprotein as a powerful tool for retrograde neuronal network tracing[J]. Neuroscience bulletin, 2020, 36: 202-216.
2. Han Z, Luo N, Ma W, et al. AAV11 enables efficient retrograde targeting of projection neurons and enhances astrocyte-directed transduction[J]. Nature Communications, 2023, 14(1): 3792.
3. Luo N, Lin K, Cai Y, et al. Microglia-specific transduction via AAV11 armed with IBA1 promoter and miRNA-9 targeting sequences[J]. bioRxiv, 2024: 2024.07. 09.602653.
The viral products used in this study, available from Brain Case, please contact bd@ebraincase.com for more information.